Duration: 24 months
This project targets flow-driven design of ablative materials, in pursuit of sustainable space missions. While necessary to preserve the equipment and the crew on board, the ablation process results in strong interactions between the surface and the surrounding air (gas-surface interaction, GSI). These interactions can have an order one influence on flow instabilities and transitions, which in turn can lead to temperature spikes and expose unprotected areas of the vehicle to reentry temperatures beyond the design values with devastating consequences. As a result, characterising GSI-effects on flow dynamics is becoming increasingly important in hypersonic research. GSI-effects can be accurately modelled by accounting for finite-rate chemistry. This approach while accurate increases the cost of base-line calculations dramatically. The excessive cost is the main reason why such interactions have not been addressed fully or with the proper level of fidelity. Instead, low-fidelity models, such as efficiency coefficients, are used, which prove insufficient for an accurate and reliable prediction of flow dynamics and maximal thermal loads. In this project, we aim to push beyond the state-of-the-art approaches in the simulation and modeling of unsteady hypersonic flight by accounting for these physicochemical effects. In order to address the excessive cost associated with the physics-based models, we will combine techniques from optimization, nonlinear model reduction, network clustering and manifold embedding to extract efficiently and adaptively a data-driven alternative, which will ultimately replace the physics-based model, reducing the overall cost while maintaining accuracy. This cost-effective data-driven model will enable the inclusion of GSI-effects into high-fidelity simulations and allow the quantitative assessment of ablative materials under reentry conditions to ultimately avoid unexpected performance of heat shields such as that of Orion Spacecraft recently.
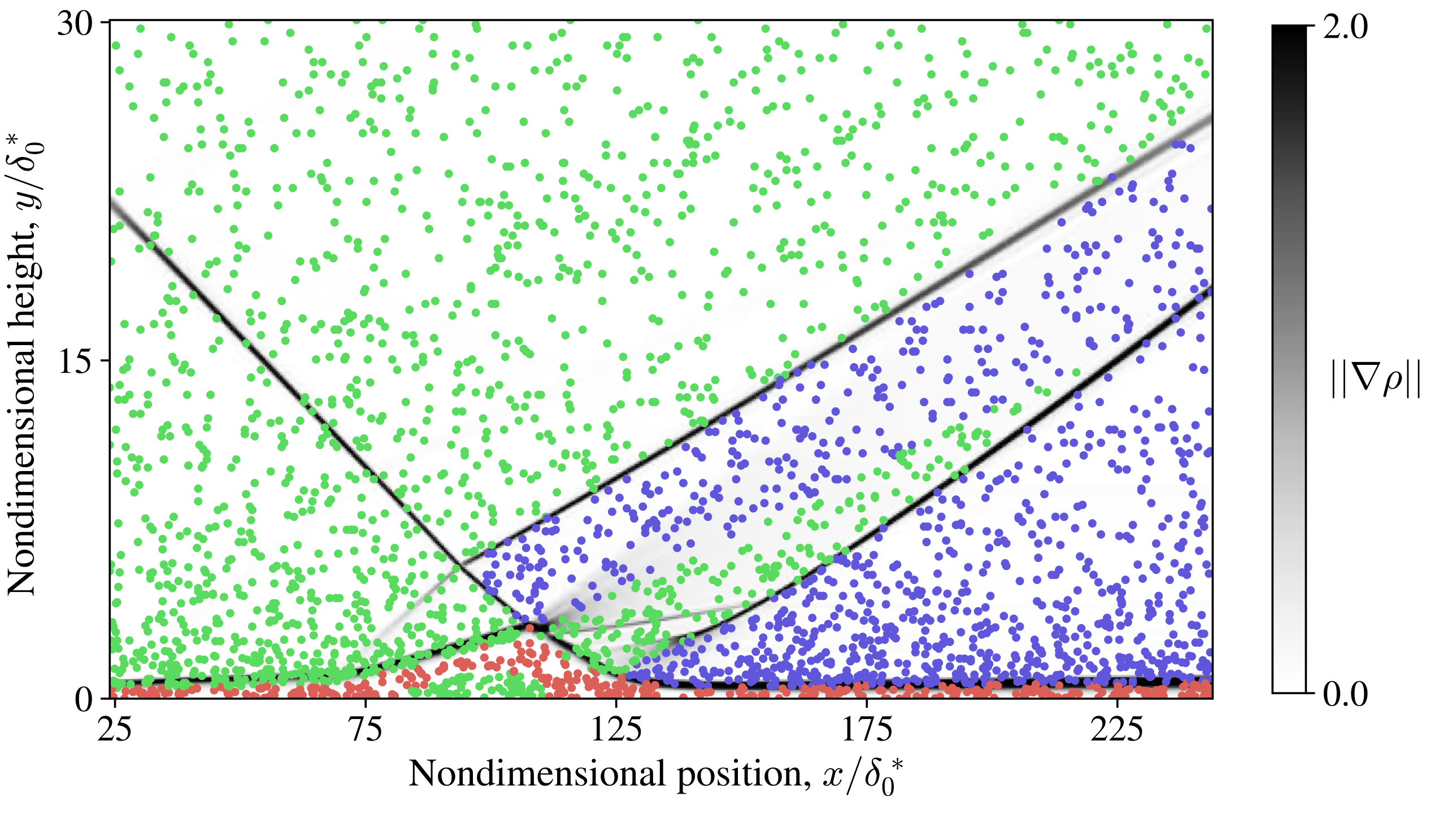