Current state-of-the-art cryocooling technologies are key enablers for a range of Earth Observation applications and Science Missions, by allowing maximum thermal noise reduction of onboard sensors. However, the main requirements for cryocoolers in almost any space application – high electrical-to-thermal efficiency, producing minimal to zero vibrations, miniaturization of the cooler and high reliability – are not met by any of today’s cryocooling methods. For instance, one of the main limitations of mechanical cryocoolers is the generation of microvibrations, which limit the optical imaging resolution of today’s Earth Observation focal plane arrays. Other main applications include the cooling of ultra-stable optical systems for next generation Science Missions. To meet all the above requirements, the novel approach of optical cryocooling has great potential, as witnessed by today’s results of generating cooling temperatures below 90 K by using the special approach of Yb3+ doped fluoride crystals co-doped with Tm3+ ions, developed at the University of Pisa (UPisa). In order for this technology to be fully competitive with existing cryocooler technology for implementation into next generation space missions, it is crucial to demonstrate the potential for further improvements in overall efficiency and cooling power. Building on a previous ESA ITI type A project performed by Lumium and UPisa, the main objective of the proposed activity is to validate a promising optical cryocooler concept that combines two advantageous features to significantly improve performance in terms of wallplug efficiency and cooling power: the use of (1) Ho3+ cooling media, and (2) an intracavity laser cooling architecture. Specifically, these advantages include (1) higher intrinsic cooling efficiency afforded by Ho-doped cooling media; and (2) optimal cooling media pumping afforded by the flexibility in cooling medium geometry, pumping beam parameters and wavelength in an intracavity scheme.
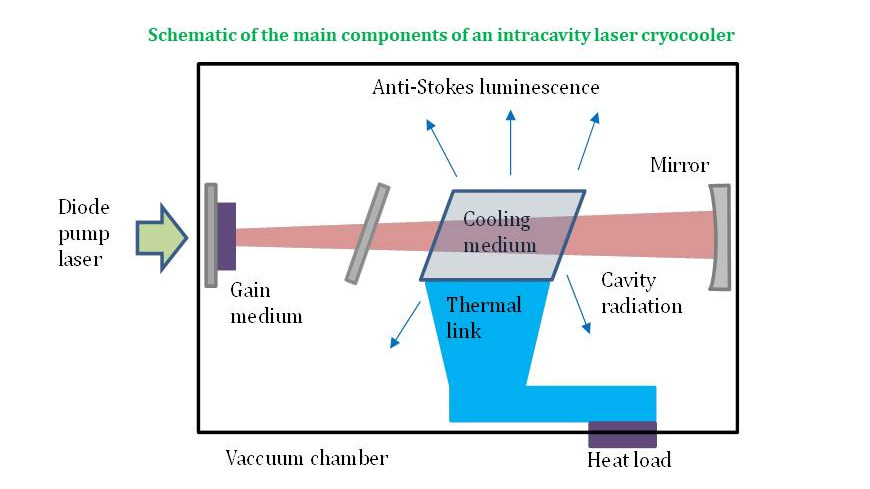