Light-pulse atom interferometers have been used to measure inertial forces and fundamental constants, test fundamental laws of physics, and search for physics beyond the standard model. Gravimeters based on atom interferometry are among the most accurate tools for measuring gravity. Transportable atomic gravimeters are being developed towards metrology, airborne sensing, shipborne surveys, and field applications. Quantum gravimeters are thus of interest as they are drift free and do not need any calibration. Their resolution improves with the square of the extended free fall, only accessible in space. Today methods exist which allow exploiting macroscopic times of tens of seconds with interferometers based on Bose-Einstein condensates. Missions such as GRACE and GOCE equipped with quantum sensors could reach a higher sensitivity and better long-term stability. A main improvement on the sensitivity shall come from the intrinsic noise of the interferometer. For this, Heisenberg limit is a way that has not been explored or implemented for field experiment. The goal of the cold atom interferometer is to measure the gravity gradient as precise as possible, but the measurement is limited by the signal to noise ratio SNR of the final detection stage. So far only uncorrelated atom clouds consisting of N atoms have been considered, which are limited in phase by the standard quantum limit N^(-1/2). Creating quantum correlations between the atoms can reduce the uncertainty, potentially reaching the Heisenberg limit of 1/N. With typically 10^6 atoms in a cloud, the sensitivity can reach 3 orders of magnitude better which leads to unprecedented inertial sensitivity. Thus these performances can be used for either gravity mission, long term space exploration or gravitational detection.
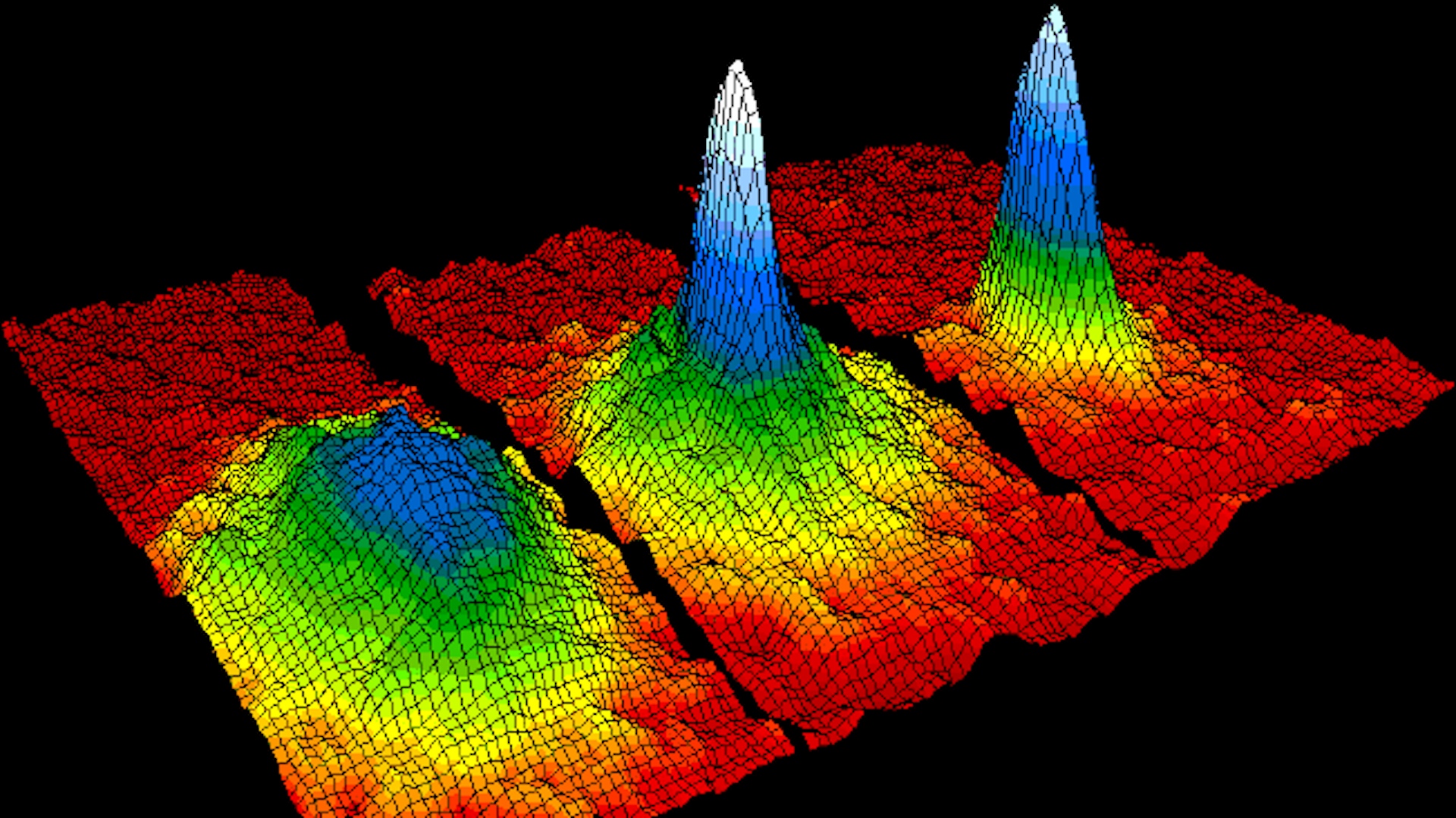