Duration: 36 months
In recent years, the interest of using nuclear fission reactors for spaceflight has again increased. Lunar surface activity is expected to intensify in the coming years due to NASA’s Artemis programme, ESA’s Terrae Novae strategy or the International Lunar Research Station. The demand for continuous and reliable power on the lunar surface will therefore increase by orders of magnitude. Nuclear reactors can be built compact [1], while supplying large amounts of power over a long period of time. Their independence of external energy sources, like solar, increases mission robustness and flexibility and enables long-term missions to the outer solar system. The increased reliability is of great interest to long-term crewed bases, where safety of the astronauts is of utmost importance, while allowing for an unrestricted operation of the base, even during a 14-day lunar night. Such missions will employ ISRU techniques to supply consumables and propellant [2], which often rely on high-temperature processes [3, 4]. Until now, nuclear reactors in spaceflight have only been considered for electric power generation, but a reactor can also supply process heat directly to the ISRU process. The high temperatures allow for a subsequent electric conversion process and the heating of habitats, storages etc. This work aims at the development of a multi-physics framework that allows for a simulation-based design of nuclear fission reactors for space applications, by using state-of-the-art numerical codes, which cover the physics from the neutronics to the thermal-hydraulics incl. ISRU and base facilities. Using the example of a crewed lunar mission, a high-temperature reactor design is proposed for heating of a molten salt electrolysis ISRU plant and crew habitats as well as electric power conversion. This work may lay a basis for a tool that enables a fast development of new reactors for future ESA missions to benefit from already existing expertise in nuclear technology across Europe.
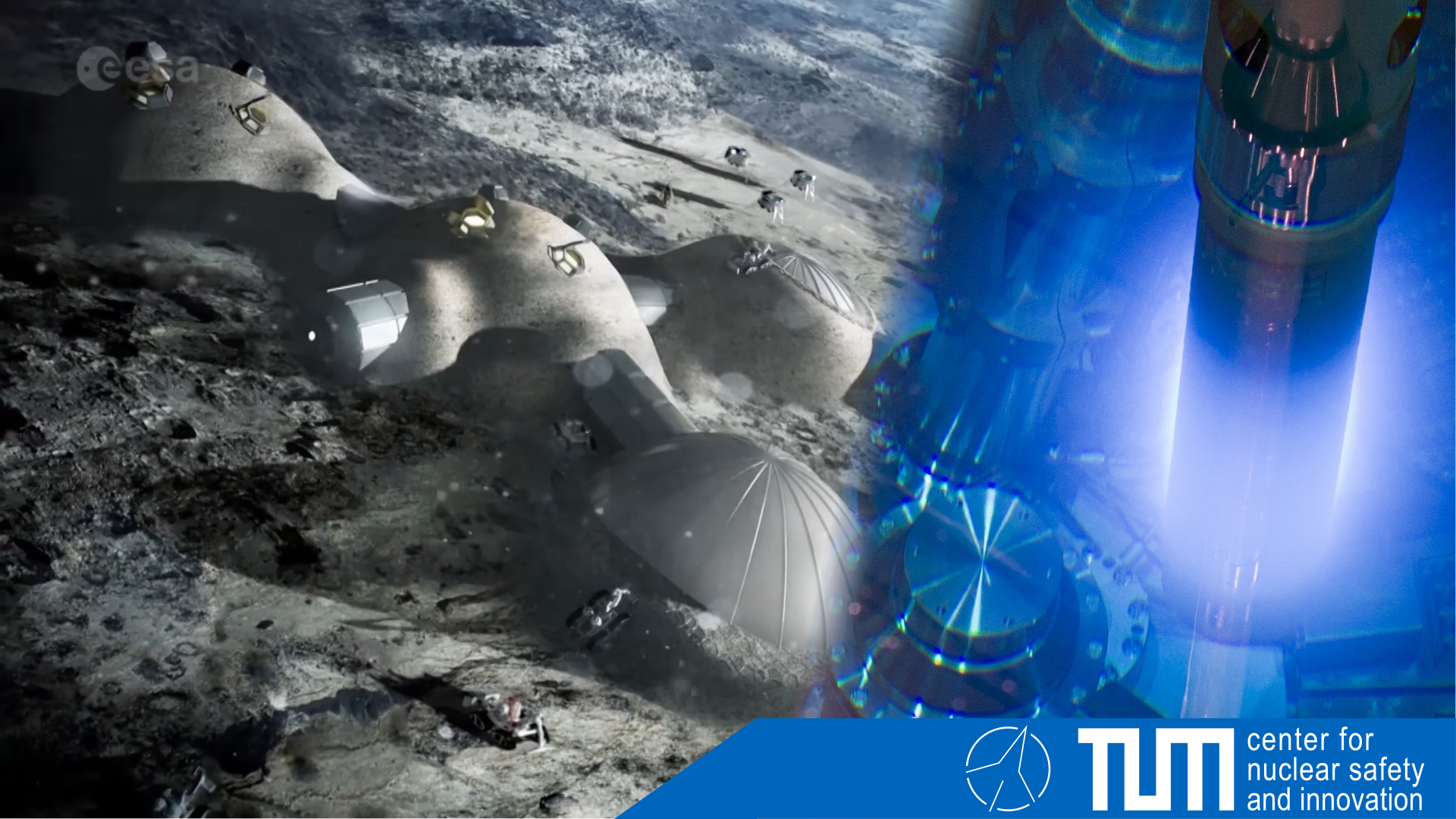